mirror of
https://github.com/youwen5/site.git
synced 2025-01-18 13:02:08 -08:00
190 lines
5.4 KiB
Markdown
190 lines
5.4 KiB
Markdown
## Introduction
|
|
|
|
The following content is a test post for the blog's markdown and $\KaTeX$ rendering capabilities.
|
|
There's a few errors, and wikilinks aren't supported. This file was copy pasted directly out of my
|
|
`obsidian.md` notebook, so it contains some weird formatting.
|
|
|
|
<br />
|
|
|
|
The methods in [[9.8 Power Series]] and
|
|
[[9.9 Representation of (Rational) Functions by Power Series]] allow us to find power series for
|
|
rational functions, $\ln$, and $\arctan$. To get power series for other elementary functions, we
|
|
need a more general method.
|
|
|
|
We can approximate some non-polynomial functions by constructing a polynomial with the _same
|
|
derivatives_ as the function. This is called a _Taylor Polynomial_.
|
|
|
|
<!-- prettier-ignore -->
|
|
> [!NOTE]
|
|
> In general, if $c \neq 0$, it's called a Taylor Polynomial. If $c = 0$, then it's a
|
|
> Maclaurin Polynomial.
|
|
|
|
<!-- prettier-ignore -->
|
|
> [!CAUTION]
|
|
> test lorem ipsum dolor sit amet consectetur adipiscing elit sed do eiusmod tempor
|
|
> incididunt ut labore et dolore magna aliqua. Ut enim ad minim veniam quis nostrud exercitation
|
|
> ullamco laboris nisi ut aliquip ex ea commodo consequat. Duis aute irure dolor in reprehenderit in
|
|
> voluptate velit esse cillum dolore eu fugiat nulla pariatur.
|
|
|
|
<!-- prettier-ignore -->
|
|
> [!WARNING]
|
|
> test lorem ipsum dolor sit amet consectetur adipiscing elit sed do eiusmod tempor
|
|
> incididunt ut labore et dolore magna aliqua. Ut enim ad minim veniam quis nostrud exercitation
|
|
> ullamco laboris nisi ut aliquip ex ea commodo consequat. Duis aute irure dolor in reprehenderit in
|
|
> voluptate velit esse cillum dolore eu fugiat nulla pariatur.
|
|
|
|
<!-- prettier-ignore -->
|
|
> [!TIP]
|
|
> test lorem ipsum dolor sit amet consectetur adipiscing elit sed do eiusmod tempor
|
|
> incididunt ut labore et dolore magna aliqua. Ut enim ad minim veniam quis nostrud exercitation
|
|
> ullamco laboris nisi ut aliquip ex ea commodo consequat. Duis aute irure dolor in reprehenderit in
|
|
> voluptate velit esse cillum dolore eu fugiat nulla pariatur.
|
|
|
|
<!-- prettier-ignore -->
|
|
> [!IMPORTANT]
|
|
> test lorem ipsum dolor sit amet consectetur adipiscing elit sed do eiusmod tempor
|
|
> incididunt ut labore et dolore magna aliqua. Ut enim ad minim veniam quis nostrud exercitation
|
|
> ullamco laboris nisi ut aliquip ex ea commodo consequat. Duis aute irure dolor in reprehenderit in
|
|
> voluptate velit esse cillum dolore eu fugiat nulla pariatur.
|
|
|
|
Consider $f(x) = e^x$. Let's find the best cubic approximation $P_3(x)$ for $f(x)$ at $c=0$.
|
|
|
|
$$
|
|
P_3(0) = f(0)
|
|
$$
|
|
|
|
$$
|
|
f'(x) = e^x,\,P_3'(0)=f'(0)
|
|
$$
|
|
|
|
$$
|
|
f''(x) = e^x,\,P_3''(0) = f''(0)
|
|
$$
|
|
|
|
$$
|
|
f'''(x) = e^x,\,P_3'''(0) = f'''(0)
|
|
$$
|
|
|
|
$$
|
|
f(0) = f'(0) = f''(0) = f'''(0) = 1
|
|
$$
|
|
|
|
We know that $P_3(x)$ will be of the form
|
|
|
|
$$
|
|
P_3(x) = a_0 + a_1 x + a_2 x^2 + a_3 x^3
|
|
$$
|
|
|
|
We can differentiate both sides $n$ times to find the values of $a_0$, $a_1$, $\dots$, $a_n$.
|
|
|
|
$$
|
|
P_3(x) = 1 + x + \frac{1}{2} x^2 + \frac{1}{6} x^3
|
|
$$
|
|
|
|
You can confirm that this polynomial has the same first, second, and third derivatives as $e^x$.
|
|
|
|
## Generalizing
|
|
|
|
Taking repeated derivatives like this leads to a common pattern in all Taylor polynomials.
|
|
|
|
<!-- prettier-ignore -->
|
|
> [!NOTE]
|
|
> We use the notation $P_n(x)$ to denote the $n^{th}$ Taylor polynomial
|
|
|
|
Taylor polynomials take the form:
|
|
|
|
$$
|
|
P_n(x) = a_0 + a_1 (x-c) + a_2(x-c)^2 + a_3 (x-c)^3 + \dots + a_n(x-c)^n
|
|
$$
|
|
|
|
where $a_n$ is the coefficient of the $n^{th}$ term (indexed from 0). It turns out that these
|
|
coefficients are actually common across Taylor polynomials.
|
|
|
|
$$
|
|
P_n(c) = a_0 = f(c)
|
|
$$
|
|
|
|
$$
|
|
P_n'(c) = a_1 = f'(c)
|
|
$$
|
|
|
|
$$
|
|
P_n''(c) = 2 a_2 = 2!\,a_2
|
|
$$
|
|
|
|
$$
|
|
P_n'''(c) = 6a_3 = 3!\,a_3 = f'''(c)
|
|
$$
|
|
|
|
$$
|
|
P_n^{(n)}(c) = n!\,a_n = f^{(n)}(c)
|
|
$$
|
|
|
|
$$
|
|
\implies a_n = \frac{f^{(n)}(c)}{n!}
|
|
$$
|
|
|
|
## Tying up loose ends
|
|
|
|
We've seen Taylor and Maclaurin polynomials. We will eventually extend them to
|
|
[[9.10 Taylor and Maclaurin Series|Maclaurin and Taylor series]]. Before this is mathematically
|
|
valid (at least, valid enough), we need to do some stuff first.
|
|
|
|
We can use Maclaurin and Taylor polynomials (or series) to estimate the value of functions by hand.
|
|
|
|
### Taylor Remainder
|
|
|
|
If you have $f(x)$ and $P_n(x)$ (centered at $c$), then $f(x) = P_n(x) + R_n(x)$, where $R_n$ is
|
|
|
|
$$
|
|
R_n(x) = \frac{f^{(n+1)}(z)}{(n+1)!}\,(x-c)^{n+1}
|
|
$$
|
|
|
|
$$
|
|
z \in [c,\,x]
|
|
$$
|
|
|
|
<!-- prettier-ignore -->
|
|
> [!TIP]
|
|
> This is a fancy way of saying that $z$ is between $c$ and $x$.
|
|
|
|
$$
|
|
\text{Error} = \left|R_n(x)\right|
|
|
$$
|
|
|
|
<!-- prettier-ignore -->
|
|
> [!NOTE]
|
|
> $R_n$ would be the "next term" in $P_n(x)$ except we put $z$ instead of $c$.
|
|
|
|
#### Applying to $e^x$
|
|
|
|
How many terms of the Maclaurin Polynomial for $f(x) = e^x$ do we need to guarantee that our
|
|
estimate for $f(1) = e$ is within $\displaystyle\frac{1}{1000000}$? The error is
|
|
|
|
$$
|
|
|R_n(1)| = \frac{|f^{(n+1)}(z)|}{(n+1)!}\,\cancel{|1-0|^{n+1}}
|
|
$$
|
|
|
|
for some $z$ between $c=0$ and $x=1$ So we want an $n$ such that
|
|
|
|
$$
|
|
\frac{|e^z|}{(n+1)!} \le \frac{1}{1000000}
|
|
$$
|
|
|
|
The worst case scenario is $z=1$. If $\displaystyle\frac{e}{(n+1)!} \le \frac{1}{1000000}$, we're
|
|
all set.
|
|
|
|
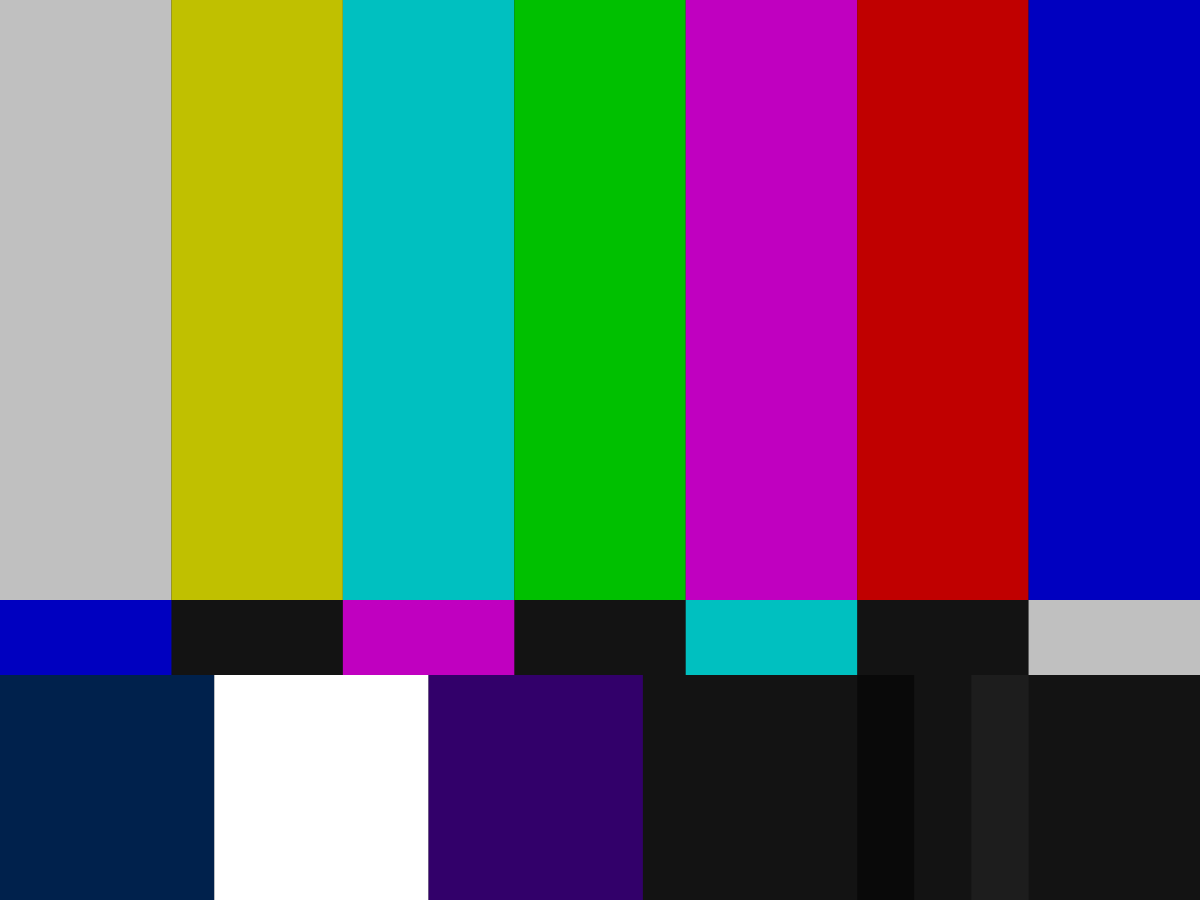
|
|
|
|
Assume that $e \le 3$, which implies that if $\displaystyle\frac{3}{(n+1)!} \le \frac{1}{1000000}$,
|
|
we're all set. After trying terms, we find that $n=9$ works. So
|
|
$P_9(1) = 1 + 1 + \frac{1}{2!} + \frac{1}{3!} + \dots + \frac{1}{9!}$ is within $\frac{1}{1000000}$
|
|
of $e$.
|
|
|
|
```rust
|
|
let mut var: u32 = 10030;
|
|
|
|
fn takes_var(var: &mut u32) {
|
|
var += 1;
|
|
}
|
|
```
|